These flexible solar cells bring us closer to kicking the fossil-fuel habit
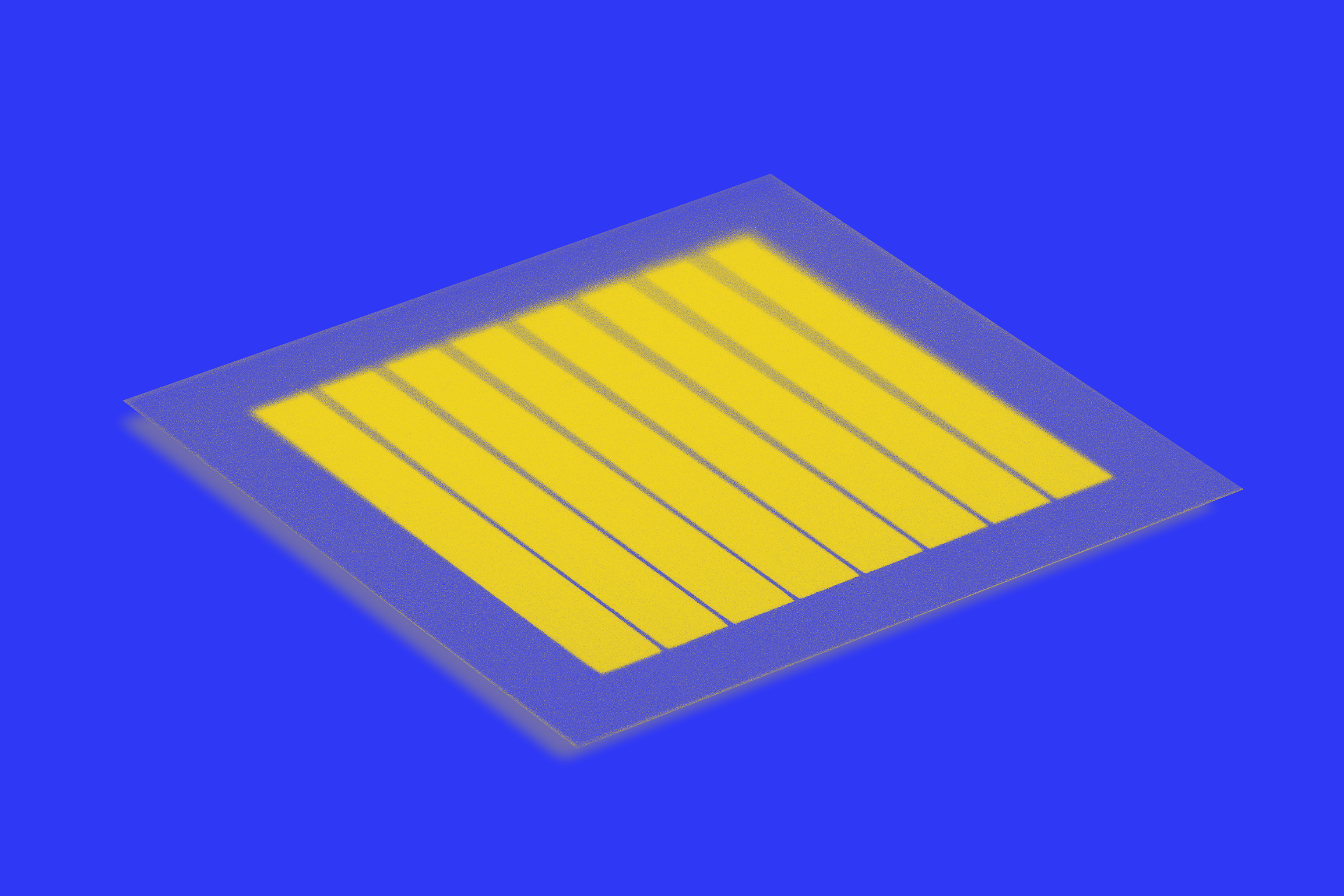
- Perovskite solar cells can be cheaper, lighter, more energy-efficient, and easier to produce than traditional silicon
- They could be put on windows, irregularly shaped surfaces, or even moving vehicles and open up a whole new range of uses for solar power, like desalination
- The biggest challenge: making them durable enough
Last December, researchers in a lab in Oxford, England, shined a sun lamp onto a tiny solar cell, only about one centimeter square.
The device was actually two cells, stacked one atop the other. The bottom one was made of the type of silicon used in standard solar panels. But the top was perovskite, a material with a crystal structure that’s particularly adept at turning light into electricity.
A pair of probes attached to the so-called tandem solar cell measured its performance. Other researchers in the lab at Oxford PV, a company spun out of the university in 2010, gathered behind a flat-screen monitor, waiting expectantly for a calculation of the cell’s efficiency to appear. When it did, they exchanged high fives. The cell had converted 28% of the energy in the light into electricity, a new efficiency record for a perovskite-on-silicon device. An independent test confirmed it a few days later, after the tiny cell was put on a plane to the National Renewable Energy Laboratory (NREL) in Golden, Colorado.


Oxford PV's commercial-sized solar cell (left), and the one-centimeter-square version (right).
While silicon panels might dominate the market—with around 95% market share—silicon is not an especially good solar material. It mainly uses light from the red and infrared end of the solar spectrum, and it has to be fairly thick and bulky to absorb and convert photons. The most efficient silicon solar panels on the market achieve less than 23% efficiency, while the theoretical maximum for a single layer of silicon is around 29%.
Perovskite, on the other hand, can use more of the light that reaches it and can be tuned to work with different parts of the spectrum. Oxford PV has opted for the blue end. Paired in a cell, the two materials can convert more photons into electrons together than either can deliver on its own.
Oxford PV plans to deliver solar cells based on perovskite and silicon to the market by the end of next year, using a German factory it acquired in 2016 from Bosch Solar. The two materials will come in a package that otherwise looks, ships, and installs the same way as a standard solar panel, in a kind of half step that the company believes will make it easier to introduce the technology to the market.

“It’s technology disruption without the business disruption,” says Chris Case, Oxford PV’s chief technology officer.
Dozens of startups that had sought to supplant silicon about a decade ago wound up in bankruptcy instead or were relegated to a niche market. But venture capitalists have invested tens of millions of dollars into perovskite ventures in recent months, heating up what had long been a frosty market for alternative solar materials. The question now is whether perovskites will fizzle too, or will finally beat silicon panels in the marketplace.
“There’s a whole set of things that make it a potentially transformational technology,” says Joe Berry, who leads the perovskite research program at the National Renewable Energy Laboratory. “But the list of technologies that have tried to compete with silicon is long and distinguished, so you have to be humble in that sense too.”
“A solar cell on steroids”
In the late 2000s, a number of well-funded startups attempted to commercialize new and more flexible solar materials, including thin-film technologies like cadmium telluride and copper indium gallium selenide (remember Solyndra?), as well as things like organic solar cells. The promise was that cells made from such materials would be far cheaper to manufacture and could be produced in various shapes.
But silicon solar panels were a fast-moving target. Efficiency levels continued to improve and prices plummeted, thanks to government-funded research efforts, market stimulation policies, and economies of scale.
Commercial photovoltaic system costs (US dollars per watt of direct current for fixed-tilt systems)

China, in particular, employed aggressive subsidies and strategies to accelerate manufacturing and exports in a quest to dominate the market. The nation’s module shipments and global market share took off starting in the mid-2000s, prompting allegations of illegal dumping aimed at knocking out overseas rivals. Prices for commercial silicon panels dropped by more than half from 2010 to 2013, and the market for alternatives sank.
So these days, to justify the vast expense of building new factories, supply chains, and distribution channels, any new material has to be better in crucial ways: more efficient, cheaper to manufacture, more versatile, longer lasting, or ideally all of the above.
Perovskite shines in some of those categories. A single layer can theoretically reach 33% efficiency, while a tandem perovskite-on-silicon device could achieve around 43%. High efficiency matters because you can produce more electricity from the same number of panels, or the same amount with a smaller footprint and lower costs.
Perovskite solar modules should also be cheaper to make, at least eventually. Producing silicon panels is a multi-step fabrication process that entails refining the silicon under high heat, infusing it with other materials, and precisely slicing it into wafers that must then be precisely patterned in a clean room to create a photovoltaic cell.
Perovskites, on the other hand, can be produced at low temperatures and used in liquid form to coat flexible materials like plastic, enabling a roll-to-roll manufacturing process similar to newspaper printing.
By repurposing Bosch’s thin-film manufacturing plant, Oxford PV expects to be able to mass-produce silicon-and-perovskite cells by the end of next year, and package them together into standard-looking panels.
“It’s an ordinary solar cell on steroids,” Case says.
In March, Oxford PV said it had raised more than $40 million to get its products into the market, bringing its total funding and financing to around $100 million. The factory will pump out 250 megawatts’ worth of cells every year.
Another perovskite startup, Energy Materials, is also looking to use roll-to-roll manufacturing. Based in Rochester, New York, it’s using film equipment originally built for Eastman Kodak to mass-produce perovskite-only solar panels. At full scale, the process will cost half as much as manufacturing a traditional solar module, while the capital costs will run an order of magnitude cheaper, because silicon requires costly, precise machines and plants, says Thomas Tombs, the company’s chief technology officer.

Since perovskite can be flexible, semitransparent, and lightweight, it could also be used where heavy, rigid solar panels wouldn’t work—on windows, creakier rooftops, irregularly shaped surfaces, or even moving vehicles.
Swift Solar, a NREL-affiliated startup that has raised nearly $7 million in recent months, is looking at putting perovskite-perovskite tandem solar cells—which use two perovskite layers, each tuned to a different part of the spectrum—on drones and electric vehicles to extend their range, according to its CEO, Joel Jean. Such a cell could be highly efficient, as well as more flexible and lightweight than one with a thick silicon layer.
Unlocking new uses for solar power
In his book Taming the Sun, Varun Sivaram, chief technology officer at ReNew Power, argues that new solar technologies like perovskites may be essential for ultimately displacing fossil fuels.
But why do we need even cheaper solar power if silicon panels are already competitive with, say, a coal plant?
One of the biggest problems with solar is that once it’s generating a significant portion of the electricity on the grid, the additional value of the next panel or plant begins to drop off sharply.
That’s because at night, solar farms don’t generate electricity at all, meaning the rest of the system still needs to be capable of meeting total demand. On sunny days, on the other hand, there may be more electricity than the system can use or store. That’s already happening in regions with lots of solar power, like Germany, China, and California.
Grid operators regularly have to force or incentivize solar farms to throttle back their production, often by pushing prices down to zero or even below. That can squeeze the solar plants’ profits, which eliminates the economic incentives to build more of them and continue reducing the use of fossil fuels.
In a paper published in Nature Energy in 2016, Sivaram and Shayle Kann, now managing director of private equity firm Energy Impact Partners, calculated that to preserve the economic incentives to keep building more plants, the cost of developing solar would need to fall to 25 cents per watt. The all-in costs of the cheapest commercial systems are $1.06 per watt, according to the latest NREL report.
Much of that is due to the high price of installing and wiring the bulky hardware. So cutting the price that dramatically will likely require not only dirt-cheap solar cells but also lightweight, flexible ones that are easier to deploy. Perovskites are the most promising material for making anything like that leap today, Sivaram says.
Cheap solar electricity could also drive down the cost of things like seawater desalination, artificial trees that can pluck carbon dioxide from the atmosphere, or electrolysis plants that can convert surplus energy into hydrogen fuel.
“It unlocks all these other new applications we never thought about before,” Sivaram says.
The instability problem
The tougher question surrounding perovskites is their durability. Efficiency leaps don’t much matter if the material lasts only a few months or even years—and so far, perovskites have tended to degrade quickly when exposed to ultraviolet light and moisture.
That’s a very big problem for a material that needs to lie under the sun for a few decades. And if companies roll out perovskite panels that end up failing too soon, it will tarnish the material’s reputation even if they later develop more durable versions.

For now, Oxford PV’s market plan depends on whether its perovskite-silicon cell can be made to work and look like a standard silicon solar panel, which includes packaging it in a glass casing that will help it last longer.
But the company did have to work hard on the stability of the material itself, employing computational modeling and rapid screening to pinpoint promising compositions among some half a million possibilities. The company’s recipe for perovskites is proprietary, but its CEO, Frank Averdung, is bullish. “We have solved the reliability issue,” he says. “We have nailed it, and this is the reason we can move into manufacturing mode now.”
Deep Dive
Climate change and energy

This rare earth metal shows us the future of our planet’s resources
The story of neodymium reveals many of the challenges we’ll likely face across the supply chain in the coming century and beyond.

Andrew Ng’s new model lets you play around with solar geoengineering to see what would happen
The climate emulator invites you to explore the controversial climate intervention. I gave it a whirl.

Want to understand the future of technology? Take a look at this one obscure metal.
Here’s what neodymium can tell us about the next century of material demand.
Stay connected
Get the latest updates from
MIT Technology Review
Discover special offers, top stories, upcoming events, and more.