This geothermal startup showed its wells can be used like a giant underground battery
If Fervo Energy’s field results work at commercial scale, it could become cheaper and easier to green the grid.
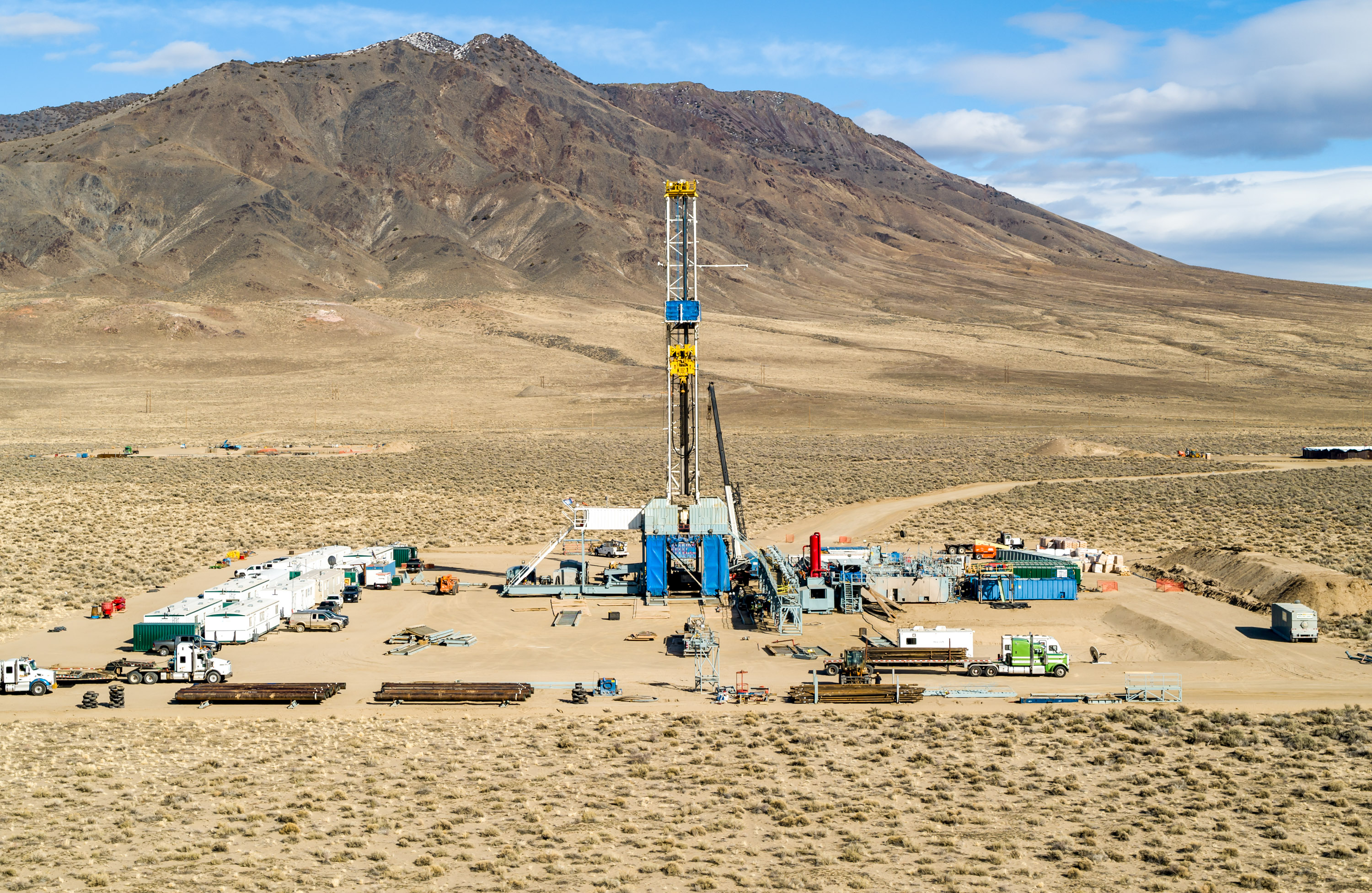
In late January, a geothermal power startup began conducting an experiment deep below the desert floor of northern Nevada. It pumped water thousands of feet underground and then held it there, watching for what would happen.
Geothermal power plants work by circulating water through hot rock deep beneath the surface. In most modern plants, it resurfaces at a well head, where it’s hot enough to convert refrigerants or other fluids into vapor that cranks a turbine, generating electricity.
But Houston-based Fervo Energy is testing out a new spin on the standard approach—and on that day, its engineers and executives were simply interested in generating data.
The readings from gauges planted throughout the company’s twin wells showed that pressure quickly began to build, as water that had nowhere else to go actually flexed the rock itself. When they finally released the valve, the output of water surged and it continued pumping out at higher-than-normal levels for hours.
The results from the initial experiments—which MIT Technology Review is reporting exclusively—suggest Fervo can create flexible geothermal power plants, capable of ramping electricity output up or down as needed. Potentially more important, the system can store up energy for hours or even days and deliver it back over similar periods, effectively acting as a giant and very long-lasting battery. That means the plants could shut down production when solar and wind farms are cranking, and provide a rich stream of clean electricity when those sources flag.
There are remaining questions about how well, affordably, and safely this will work on larger scales. But if Fervo can build commercial plants with this added functionality, it will fill a critical gap in today’s grids, making it cheaper and easier to eliminate greenhouse-gas emissions from electricity systems.
“We know that just generating and selling traditional geothermal is incredibly valuable to the grid,” says Tim Latimer, chief executive and cofounder of Fervo. “But as time goes on, our ability to be responsive, and ramp up and down and do energy storage, is going to increase in value even more.”
‘Geothermal highway’
In early February, Latimer drove a Fervo colleague and me from the Reno airport to the company site.
“Welcome to Geothermal Highway,” he said from behind the wheel of a company pickup, as we passed the first of several geothermal plants along Interstate 80.
The highway cuts through a flat desert in the midst of Nevada’s Basin and Range, the series of parallel valleys and mountain ranges formed by separating tectonic plates.
The crust stretched, thinned, and broke into blocks that tilted, forming mountains on the high side while filling in and flattening the basins with sediments and water, as John McPhee memorably described it in his 1981 book, Basin and Range. From a geothermal perspective, what matters is that all this stretching and tilting brought hot rocks relatively close to the surface.
There’s much to love about geothermal energy: it offers a virtually limitless, always-on source of emissions-free heat and electricity. If the US could capture just 2% of the thermal energy available two to six miles beneath its surface, it could produce more than 2,000 times the nation’s total annual energy consumption.
But because of geological constraints, high capital costs and other challenges, we barely use it at all: today it accounts for 0.4% of US electricity generation.
To date, developers of geothermal power plants have largely been able to tap only the most promising and economical locations, like this stretch of Nevada. They’ve needed to be able to drill down to porous, permeable, hot rock at relatively low depths. The permeability of the rock is essential for enabling water to move between two human-drilled wells in such a system, but it’s also the feature that’s often missing in otherwise favorable areas.
Starting in the early 1970s, researchers at Los Alamos National Laboratory began to demonstrate that we could engineer our way around that limitation. They found that by using hydraulic fracturing techniques similar to those now employed in the oil and gas industry, they could create or widen cracks within relatively solid and very hot rock. Then they could add in water, essentially engineering radiators deep underground.
Such an “enhanced” geothermal system then basically works like any other, but it opens the possibility of building power plants in places where the rock isn’t already permeable enough to allow hot water to circulate easily. Researchers in the field have argued for decades that if we drive down the cost of such techniques, it will unlock vast new stretches of the planet for geothermal development.
A noted MIT study in 2006 estimated that with a $1 billion investment over 15 years, enhanced geothermal plants could produce 100 gigawatts of new capacity on the grid by 2050, putting it into the same league as more popular renewable sources. (By comparison, about 135 gigawatts of solar capacity and 140 gigawatts of wind have been installed across the US.)
“If we can figure out how to extract the heat from the earth in places where there’s no natural circulating geothermal system already, then we have access to a really enormous resource,” says Susan Petty, a contributor to that report and founder of Seattle-based AltaRock Energy, an early enhanced-geothermal startup.
The US didn’t make that full investment over the time period called for in the report. But it has been making enhanced geothermal a growing priority in recent years.
The first major federal efforts began around 2015, when the Department of Energy announced plans for the Frontier Observatory for Research in Geothermal Energy laboratory. Drilling at the selected Utah FORGE site, near Milford, finally commenced in 2016. The research lab has received some $220 million in federal funds to date. More recently, the DOE has announced plans to invest tens of millions of dollars more in the field through its Enhanced Geothermal Shot initiative.
But there are still only a handful of enhanced geothermal systems operating commercially in the US today.
Fervo’s bet
Latimer read that MIT paper while working in Texas as a drilling engineer for BHP, a metal, oil, and gas mining company, at a point when he was becoming increasingly concerned about climate change. From his own work, he was convinced that the natural-gas fracking industry had already solved some of the technical and economic challenges highlighted in the report.
Latimer eventually quit his job and went to Stanford Business School, with the goal of creating a geothermal startup. He soon met Jack Norbeck, who was finishing his doctoral dissertation there. It included a chapter focused on applied modeling of the Los Alamos findings.
The pair cofounded Fervo in 2017. The company has since raised nearly $180 million in venture capital from Bill Gates’s Breakthrough Energy Ventures, DCVC, Capricorn Investment Group, and others. It’s also announced several commercial power purchase agreements for future enhanced-geothermal projects, including a five-megawatt plant at the Nevada site that will help power Google’s operations in the state.
Under those deals, Fervo is contracted to provide a steady flow of carbon-free electricity, not the flexible features it’s exploring. But almost from the start, utilities and other potential customers told the company that they needed to line up clean sources that could ramp generation up and down, to comply with increasingly strict climate regulations and balance out the rising share of variable wind and solar output on the grid.
“If we can come up with a way to solve this,” Norbeck says he and Latimer realized, “we might really have a way to change the world.”
Fervo began to explore whether they could do so by taking advantage of another feature of enhanced geothermal systems, which the Los Alamos researchers had also highlighted in later experiments.
Creating fractures in rocks with low permeability means that the water in the system can’t easily leak out into other areas. Consequently, if you close off the well system and keep pumping in water, you can build up mechanical pressure within the system, as the fractured rock sections push against the earth.
“The fractures are able to dilate and change shape, almost like balloons,” Norbeck says.
That pressure can then be put to use. In a series of modeling experiments, Fervo found that once the valve was opened again, those balloons effectively deflated, the flow of water increased, and electricity generation surged. If they “charged it” for days, by adding water but not letting it out, it could then generate electricity for days.
But the company still needed to see if it could work in the real world.
The tests
After crossing in Humboldt County, Nevada, Latimer eventually steered onto a dirt road. The Fervo site announced itself with a white drilling rig in the distance, soaring 150 feet above a stretch of brown desert. The geology under this particular stretch of land includes hot rocks at shallow depths, but not the permeability needed for traditional plants.
In 2022, the company drilled twin boreholes there, using a nearly 10-inch fixed-cutter drill bit to slowly grind through mixed metasedimentary and granite formations. The wells gradually bend beneath the earth, ultimately plunging some 8,000 feet deep and running around 4,000 feet horizontally.
Fervo then injected cold water under high pressure to create hundreds of vertical fractures between them, effectively forming a giant underground radiator amid rock that reaches nearly 380 ˚F (193 ˚C).
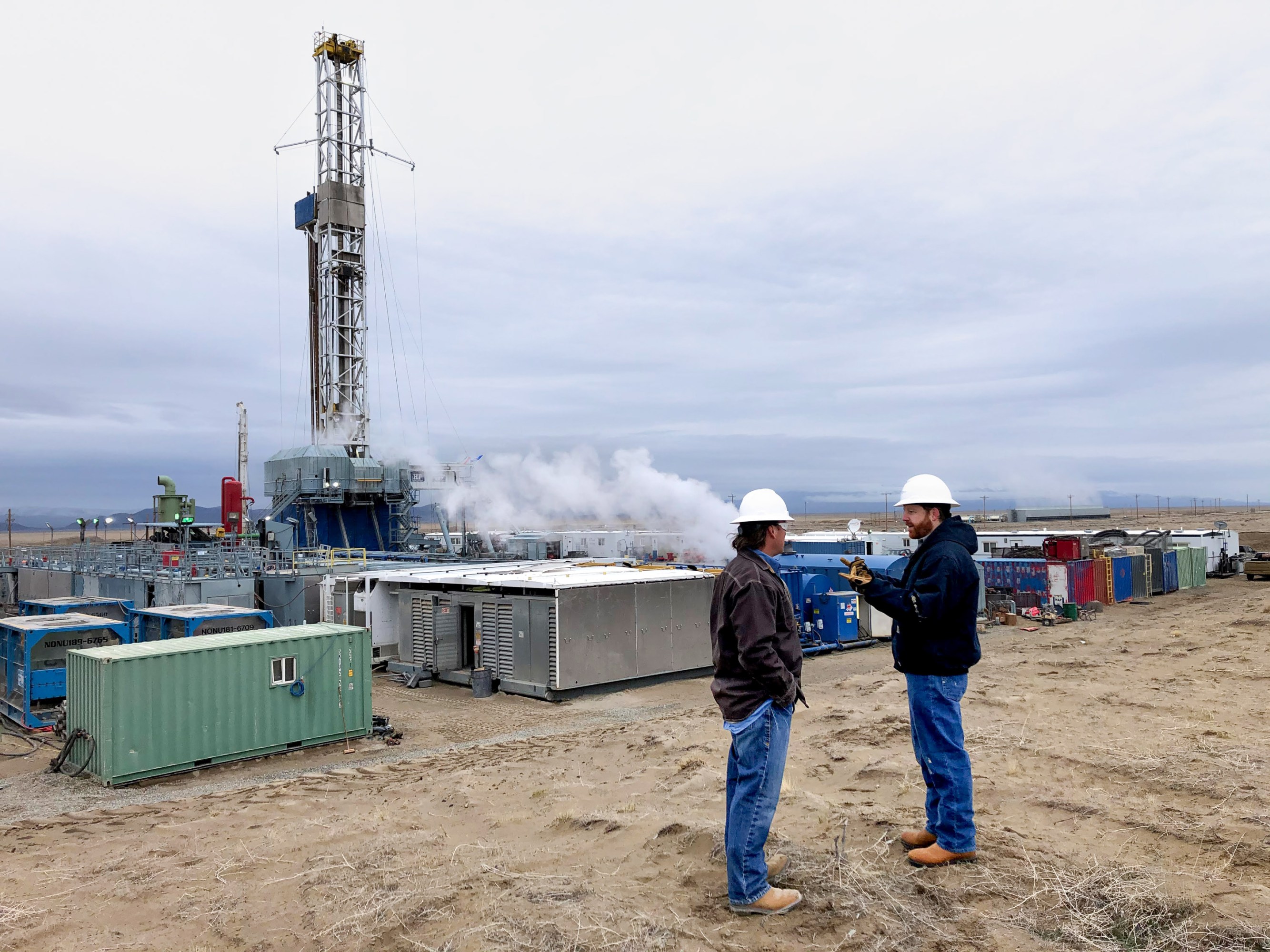
Around 8 a.m. on January 28, the company shut off the valve on what’s known as the production well, where the water would normally surface, starting the first tests of what it calls Fervo Flex. The pressure shot up to several hundred pounds per square inch and kept building gradually over the next 10 hours or so.
Norbeck was standing near that well when they opened it back up around 7 p.m., his eye trained on the bubble gauge of a big yellow weir box, a simple, time-tested tool for measuring flow rates. The hot water produced a flash of steam as it hit the open air, and the readings peaked.
Fervo's employees continued the tests for days, shutting the well down for eight to 10 hours and opening it back up for 14 or more, operating it as they would on a grid with plentiful daytime solar power. On the morning of our visit, the company was several days into an effort to operate the system without pumping in more water, to understand how long it could last as a form of energy storage.
Fervo may be the first company to field-test this means of combining storage and flexibility at an enhanced-geothermal site. The US Department of Energy’s ARPA-E division provided $4.5 million in funding for the experiments.
Inside the site’s safety trailer, Latimer opened a laptop and began clicking through a presentation. A set of charts displayed a series of smooth curves and spikes as pressure built and production soared in each of the tests. Then he clicked to a page that showed the earlier results from the models, which more or less mirrored the results.
“It works, is the punchline,” Latimer said. “What we modeled is exactly what happened.”
Value to the grid
The core challenge in creating a carbon-free power sector is that the amount of electricity generated from wind and solar farms fluctuates dramatically through the day and year.
This will create increasingly significant challenges as renewables come to dominate electricity grids. Studies find that total system costs begin to rise sharply as renewables exceed about 80% of generation—unless there are major sources of carbon-free electricity that can work on demand, cheaper forms of long-duration energy storage, or other technical solutions.
That’s because there can be extended periods of the year when solar, wind, and other fluctuating sources don’t provide enough energy to keep things running through the night or day. Regional grids relying almost entirely on those resources would often have to add massive banks of expensive and relatively short-lived batteries as well as more renewables plants to charge them, just to keep the lights on through those stretches.
A geothermal power plant that can dial electricity up and down, and fill in for waning renewables for hours to days, promises to address those challenges, providing a highly valuable resource for grids that are growing increasingly green.
“The technology innovations that we’re demonstrating … would easily enable geothermal to fill that 20% role,” Latimer says.
Last year researchers at Princeton, working with Fervo, ran a series of simulations of carbon-free electricity grids across the western US in 2045, exploring what sets of technologies would be most attractive for the lowest-cost versions of such systems.
Adding Fervo’s flexibility features made geothermal a much more appealing option. Today there’s only about four gigawatts of geothermal energy in the US. But for future scenarios, the model added between 25 and 74 gigawatts of flexible geothermal capacity to its carbon-free grids, compared to only up to 28 gigawatts when geothermal plants couldn't operate in that way. The added capability of those facilities also drove down total grid system costs by as much as 10%.
“If we can make it work … it could be a very large deal,” says Wilson Ricks, a Princeton energy systems researcher and the lead author of the working paper.
These features should also increase the economic value and profits of the geothermal plants themselves, potentially making them easier to finance.
Other companies long ago figured out ways of cranking down the output of geothermal plants. But it often doesn’t make much financial sense to do so — you’re just shutting down the plant and not getting paid.
In Fervo’s case, though, these facilities could throttle down during periods when ample solar or wind is depressing the wholesale price of electricity, and crank out more than usual when those sources decline and prices rise, Latimer says.
Open questions
Fervo still faces some real challenges, however.
While all of this looks great in models and now in field tests, making the numbers work for commercial plants might require significant changes in electricity market rules and power purchase agreements. The structures in place today still largely reward operators for cranking at max capacity at all times.
The company will also need to do much more work to demonstrate that these storage and ramping capabilities can work continuously within large-scale commercial plants operating in a variety of regions and geologies.
Meanwhile, some important questions remain about enhanced geothermal as a basic concept, leaving aside the added features Fervo is exploring.
The field suffered a serious blow in 2009, when an early commercial effort in Basel, Switzerland, appeared to trigger a series of small earthquakes, including a magnitude 3.4 event, which reportedly caused several million dollars in damages.
There have been significant advances since in site selection, well design, and other practices that minimize the possibility of inducing sizable seismic events, says Joseph Moore, the managing principal investigator at Utah FORGE. The additional storage and flexibility features Fervo is exploring shouldn’t introduce any additional dangers of this sort, he adds.
But induced seismicity remains an issue that must be handled carefully and monitored for continually, and it does create concerns for communities considering such projects.
In addition, there simply haven’t been many enhanced geothermal systems built or run over extended periods. It may still prove difficult or expensive to reliably create enough fractures and pathways to ensure the necessary flow rates in certain cases and places, says Travis McLing, the geothermal program lead at the Idaho National Laboratory.
In addition, the systems could lose permeability over time as biofilms emerge in the wells, minerals form in the fractures, and other changes occur. That could reduce the output and undermine the economics, McLing says. “Reservoir sustainability is my biggest concern,” he wrote in an email.
‘Core fundamentals’
Latimer also stresses that the geothermal field has made significant improvements in understanding seismic risks and developing practices that minimize the odds of inducing significant earthquakes.
That includes drilling horizontally through multiple geological zones to average pressure shifts across broader areas, as Fervo has done in Nevada. The company has also partnered with the US Geological Survey to closely monitor seismicity on the site and evaluate other techniques developed to further reduce such risks.
Fervo’s commercial plan is still primarily focused on producing a steady flow of clean electricity. The Nevada plant is set to begin delivering precisely that to Google and other customers later this year.
But Latimer and Norbeck believe that the flexibility and storage features will be an economic bonus on top of the core advantages of enhanced geothermal systems, and that the initial field results show it’s well worth continuing to explore the potential.
“It gave us confidence that the core fundamentals are there,” Norbeck says. “Now it comes down to optimization, cost reductions, and things like that. But the physics are all validated, and the concept can work.”
Deep Dive
Climate change and energy

This rare earth metal shows us the future of our planet’s resources
The story of neodymium reveals many of the challenges we’ll likely face across the supply chain in the coming century and beyond.

Andrew Ng’s new model lets you play around with solar geoengineering to see what would happen
The climate emulator invites you to explore the controversial climate intervention. I gave it a whirl.

Want to understand the future of technology? Take a look at this one obscure metal.
Here’s what neodymium can tell us about the next century of material demand.
Stay connected
Get the latest updates from
MIT Technology Review
Discover special offers, top stories, upcoming events, and more.